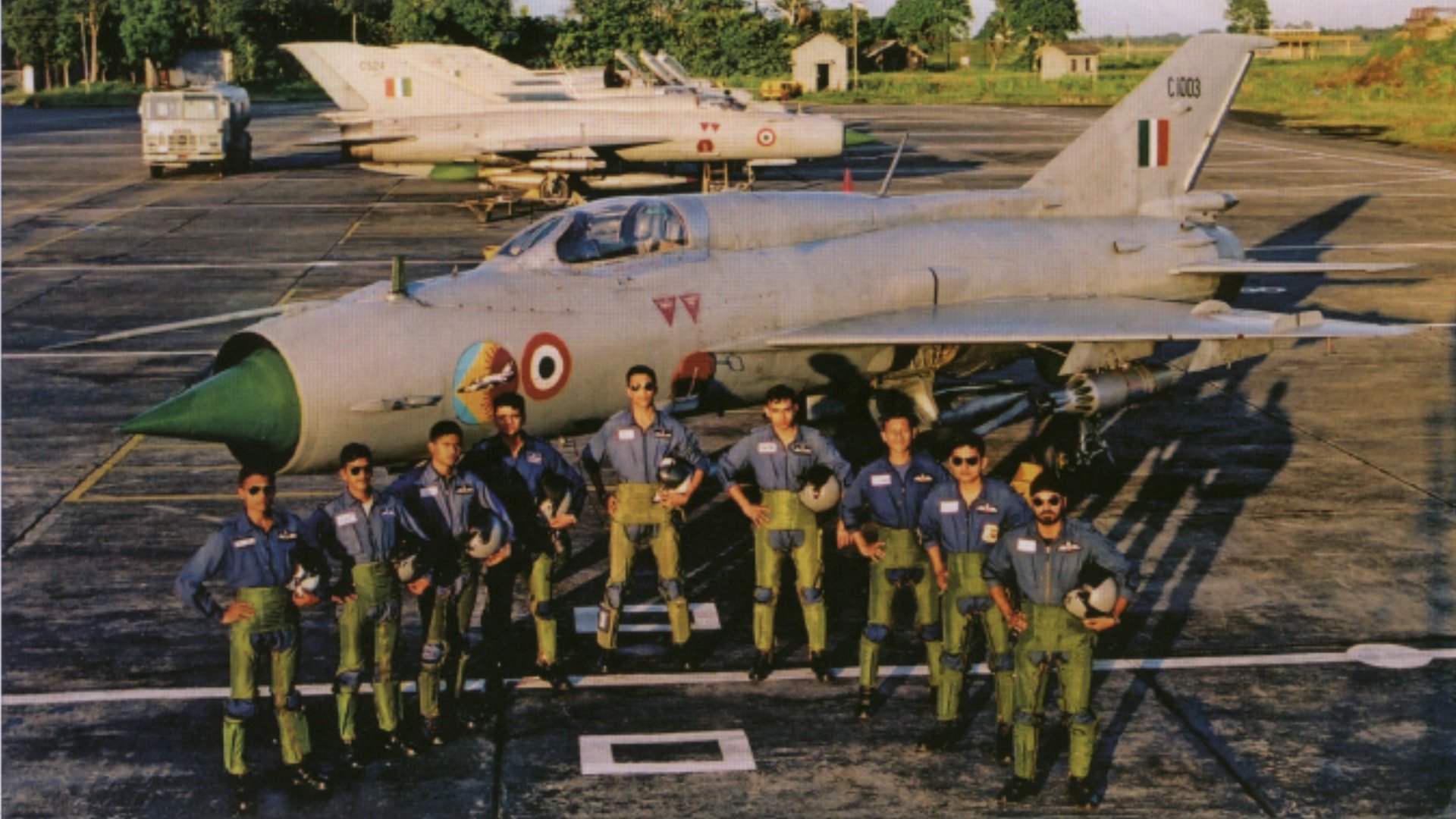
THE MIG-21 IN THE IAF: SIX DECADES OF UTILISATION
BLOGS
The concept of air warfare in the USSR post WWII was simple and utilitarian: numbers versus quality. In effect, this meant that as long as they had enough aircraft, they could afford to lose part or all of a fleet of fighters engaged in any air-related combat. Victory would ensue in time, loss of numerous aircraft notwithstanding. The enemy force would not be able to survive attrition built up on a progressive basis and necessarily withdraw. Pilots were assumed to be an expensive community in the perceived enemy, whereas the USSR could afford to lose pilots, as they were only statistical figures.
The USSR did not have a clue as to how to design aircraft, leaving it to only the Mikoyan Gurevich Design Bureau founded in 1939 by aircraft designers Artem Mikoyan and Mikhail Gurevich, and the Sukhoi Design Bureau, also founded in 1939, by Pavel Sukhoi. Aircraft designed by the former bureau would be called MiGs, with a suitable numerical appendage, like MiG-15/MiG-29 and so on. The same principle was applied across the Sukhoi bureau.
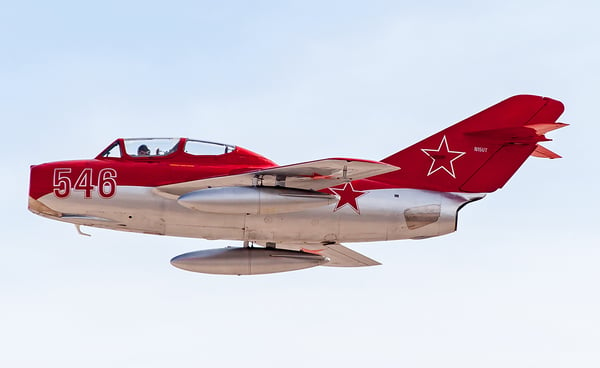
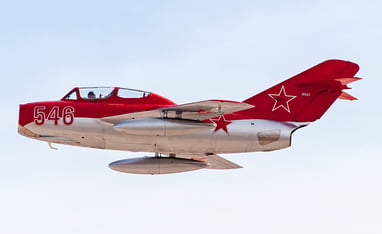
After WWII, the airframes and engines of captured or shot down enemy aircraft were closely studied to select the material to build the MiGs and the Sukhois. Misfits or poor alignment when building an aircraft were not a problem. Airframe engineers would force misaligned segments into place with wooden mallets as well as drive rivets in by force and tighten the screws on the other side using hydraulic spanners/wrenches. The importance of surface condition and effect of leading edge roughness were given short shrift.
Things improved with time, with the jet-engined MiG-15 first flying in 1947 and proving hugely successful in the Korean War. Its successor, the MiG-17 also proved its worth in the Vietnam war. 13,000 MiG-15s and nearly 11,000 MiG-17s were built. After the MiG-19, the MiG-21 of the Cold War was a product of new ideas introduced by a new school into an old workshop. In addition, there were two entities to ponder over, viz., an engine and an airframe, one within the other. Matching timelines were never considered as there were two separate design bureaus at play, the Mikoyan-Gurevich and the Tumansky (Gavrilov). This would go on to play havoc with the serviceability figures of all Air Forces that would import these aircraft in the future.
It is well known why we procured Soviet aircraft, fighters, freighters and helicopters. Most of them were third rate acquisitions, like the IL-14, Mi2, Mi4, Mi 26, SU-7 and the MiG-23, among others. The early acquisitions were paid for with bananas and Bata slippers, a straightforward barter. The MiG-21 was first flown by IAF pilots in USSR in 1962 and inducted in Sqn strength as Type 74 aircraft the next year.
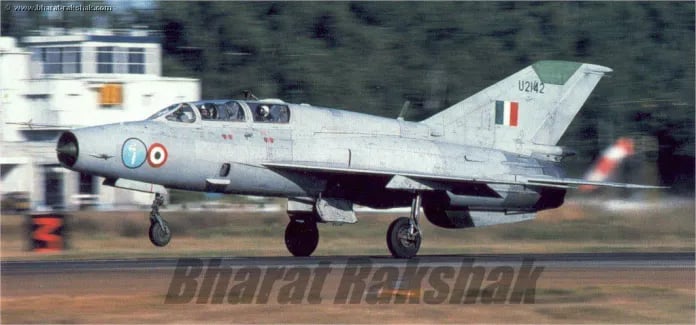
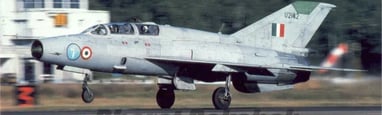
Flying the Type 74 was a nightmare. Pilots had to don pressure suits for each and every sortie, making them look like cosmonauts. It took 30 min to put them on, then an excruciating and sweaty 15 min ground delay before takeoff and climb out, since pressurisation cut in only at 2.0 km AMSL- and a 25-30 min sortie. Taking the kit off was no easy task, either. Thankfully, wiser counsel prevailed and use of these monkey suits was effectively withdrawn in the next lustrum, with the advent of the Type 76. Only sorties above 11 km required these kits, so all sorties were kept to 100m-10 km AMSL. They had to be worn mandatorily for airtest sorties, which required a supersonic run up to M 2.05 at or above 13 km. To add to their woes, the Type 74 had problems of stability in the M 0.81-0.84 range, which would continue to manifest itself, to a slightly lower degree, even in the Type 75 Bis.
The IAF has been stuck with the MiG-21 family for generations. The Type 74 and its Type 66 trainer gave way to the Type 76 with the Type 66A trainer, followed by the Type 77. The Western world called this a high speed toy; they weren’t far from the truth. Well after the initial buy, we got a Transfer of Technology. HAL Ozar was upgraded to build the Type 77 aircraft, rather than just assemble/service them. The engines came later, from HAL Koraput. The two were paired at Ozar. There was a flurry of technical failures leading to losses of aircraft immediately after the 1971 war, as the aircraft had been overstretched in that brief but intense period. HAL sent out teams to carry out repairs in situ, if they could. As the IAF advanced, intermediate trainers like the excellent Hunter were phased out due age. Pupil pilots were now moving from the HJT-16 Kiran and the Polish TS-11 Iskra to the MiG-21 Type 77 directly. Transition was very difficult for these pilots; the take-off and landing speeds of the Type 77 (340 kph) were close to the aerobatics speeds for the just-flown trainers. Stick movements were grossly exaggerated in the MiG-21 family. No surprise then that this was the phase of maximal losses due to human error.
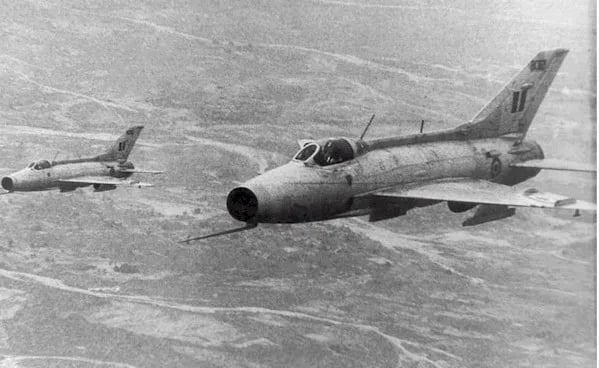
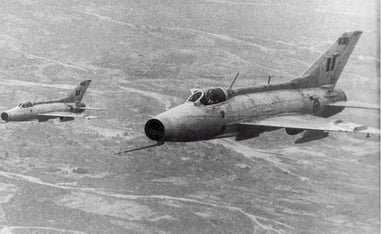
The MiG-21 was/is an unforgiving beast. Moreover, the Type 77 had an ejection seat designed for ejection at high altitude at high Mach nos. When used, the canopy would detach nose-first and as the seat rose, slap down on the sides of the seat, the rear end of which would climb into the detached rear end of the canopy, encapsulating the pilot as the drogue chute extracted the main chute. But, below 100m/300kph, the inherent procedural delay would invariably prove fatal. To add to these woes, a large number of tyro pilots were lost in their inability to recognise and react to deep stalls. The worst period was between 1994-2007, by which time enough Hawk intermediate jet trainer (IJT) aircraft were acquired- followed in 2013 by the Pilatus PC-7. The Bis/Bison had a 0/130 kph ejection minima, an acceptable set of criteria.
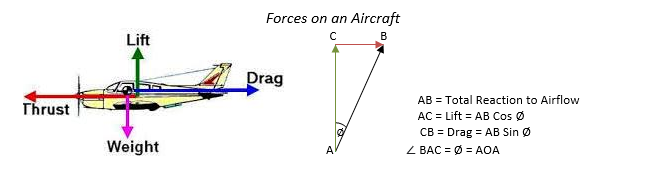
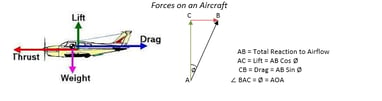
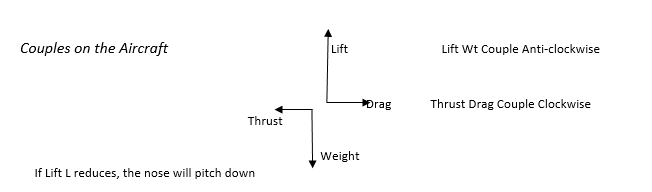
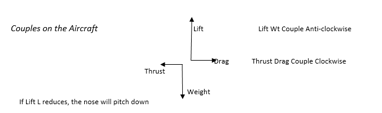
Even though intermediate trainer aircraft bridged the gap, the accident rate did not reduce as expected. There were far too many technical defects cropping up on the ageing aircraft and young pilots, oblivious to the world of near uncontrollable deep stalls in delta wing aircraft, added to the problem with uninformed actions/inaction. They had been shown and taught stalls on the IJT. At a preset throttle rating, the aircraft nose would be lifted to increase angle of attack (AOA) and also lift. AOA is the angle at which the incoming airflow meets the chord of the wing, an imaginary line from the leading to the trailing edge of the wing. Drag would also increase. These aerodynamic forces were a function of the lift and drag indices and the square of the velocity. At ~11-13°AOA, the aircraft would start to judder, implying turbulent airflow over the control surfaces, and then stall at 14-15°AOA. The quad forces acting on the aircraft in flight [L, D, Weight (W), Thrust (T)] would vary in reciprocal moment arms to maintain equilibrium. Since lift would drop to near zero at the stall, equilibrium would be lost leading to a forced nose down pitch at the stall. Recovery was simple; push down on the stick to lower the nose and AOA, open power to maximum and recover the aircraft. A simple and harmless exercise. Sadly, not so on the delta-wing MiG-21.
In a delta-wing aircraft, the airflow is required to travel faster at the wing root, which chord is multiple times longer than the chord at and near the wing tip as the swept back wing tapers laterally, and airflow separation from the wing starts from the thickest part of the wing, the wing root. This causes the airflow to deflect sideways toward the wingtip. Moreover, when and wherever the two flows (above and below the wing) meet, they create a vortex. This vortex will invariably travel out and downwards.
On the MiG-21 family, mainly the Bis and Bison, you could raise the nose up to 26-29° and the AOA gauge would show you an AOA of 26-29°. Thereafter, the aircraft would start to sink and the nose would slowly drop to ~15-16° above the horizon, but the actual AOA would keep rising, go well beyond the degree scale on the AOA marker; the aircraft would continue to sink at an increasing rate. The AOA gauge would start to flash AOA warnings at around the 23° mark, and keep flashing, but recovery was not as simple as taught earlier; there was no pitch-down either. Increasing power would help initially, but that contribution to lift would soon be less than the rise in drag. AOA could increase up to 60-80° but the nose would not drop below the horizon. Worse would follow.
Downwash
We are all aware of the serious danger an airliner can get into if it flies into the downwash of the preceding liner on approach and even take off, particularly if it is ‘Heavy’ or ‘Super’. The downwash is directly proportional to the weight of the preceding aircraft, as it is a major component of the lift generated. (L > W if you wish to get airborne and fly out). A fully controlled L < W parameter is required to allow the aircraft to descend at a calibrated rate and land. It is the same for fighter aircraft, but the only heavy fighter in the IAF is the SU-30 MKI. (I have seen a Jaguar 2 km behind a Mirage IV in France crash at ~50’). Downwash is the change in direction of air deflected by the aerodynamic action of an aerofoil and /or wing as part of the process of producing lift. The air is forced downward in a spiral behind the wing.
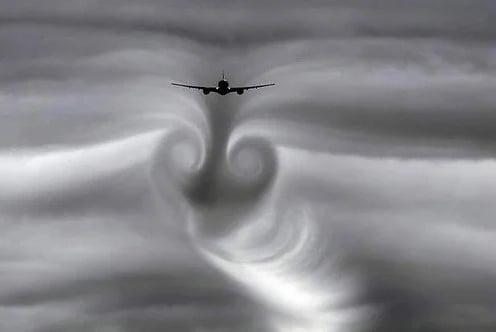
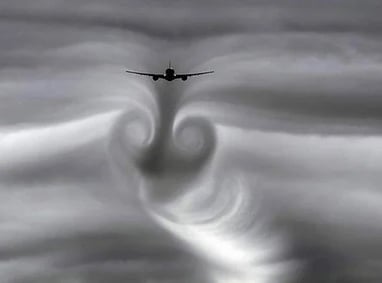
Induced Downwash
The trailing vortices modify the whole flow pattern. In particular, they alter the flow direction and speed in the vicinity of the wing and tail surfaces. The trailing vortices therefore have a strong influence on the lift, drag and handling qualities of an aircraft. Downwash also influences the flow over the wing itself, with important consequences. Firstly the angle of attack relative to the modified total airstream direction is reduced. This in effect is a reduction in angle of attack (AOA), and means that less lift will be generated unless the angle of attack is increased further, which, in turn, increases both drag and downwash! The second consequence is that the lift force vector is tilted backward relative to the free stream flow, thereby creating a rearward component of the force, vortex or induced drag. A further serious consequence of downwash is that the airflow approaching the tailplane is deflected downwards so that, initially, the effective angle of attack of the tailplane is reduced. As downwash and sideways movement of the airflow increases with AOA, the airflow that was passing over the tailplane is suddenly cut off. Now, there is no airflow over the tailplane. The aircraft is out of pitch control. There is no sudden downward movement of the nose. It will not move at all in the vertical plane! There would be little effect on the rudder.
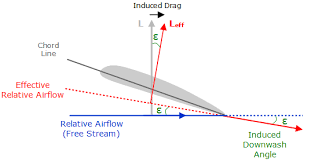
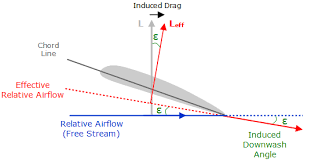
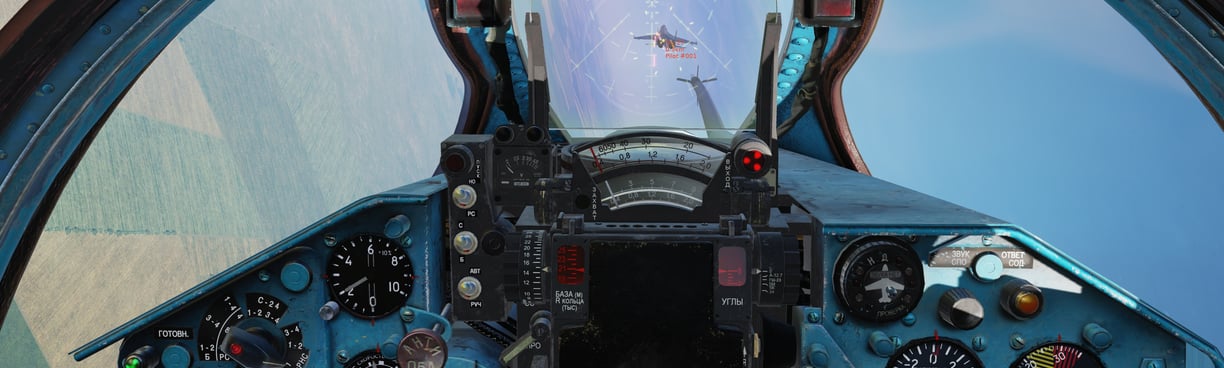
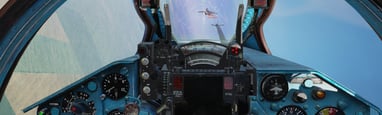
This effect is not limited to take off and landing only. It is most commonly seen in practice air combat. This is why most accidents under the pilot error category were during the exhaustive air combat training phase of the syllabus. The recommended solution to a recognised deep stall, if nothing worked, was to deploy tailchute.
This jerk would create a strong pitch down and the aircraft retrieved into the flyable zone. Yes, the tailchute cords were very strong, usable at speeds up to 360 kph on the ground, but they had to be jettisoned quickly. The USP of the Bis family was its Emergency Power Rating (EPR). In this mode, the N1 Turbine was forced to spool up to 102.5%, by adding extra fuel to that being fed into the engine combustion chamber. There is a perceptible increase in thrust, from ~70 kN to ~97 Kn and requires a deliberate decision by a pilot to engage EPR. The higher compressor blade speed would lead to an increased compression ratio, with a rise in exhaust temperature. The air density was insufficient to produce the required thrust above 7000’ AMSL and an auto-cutoff was brought into play at 2.0 Km AMSL. Use of EPR was limited to 02 min in a sortie, with the possibility of overtemp, burnout or explosion if it was used > 02 min. One minute of EPR was considered equal to 01 hour of engine life and its use was cut off totally at the 50 min stage.
The maintenance schedule of the aircraft was harrowing. If the Bis was annoying, think about the electronically advanced Bison! It was like so under ideal conditions:
1. 25 Airframe hours: One day for servicing.
2. 50 Airframe hours: Six working days for servicing. The rear fuselage is opened up. The avionics suite is also tested. Since the avionics suite was BITE tested mission to mission, the latter task is probably unrequited or relatively simple.
3. 75 Airframe hours: Same as 25 Airframe hours.
4. 100 Airframe hours: Fifteen working days for servicing. The rear fuselage is opened up. The engine is also opened and parts tested. The effect of EPR would be assessed. The avionics suite would be tested to its limits.
5. 125 Airframe hours: Same as 25 Airframe hours.
6. 150 Airframe hours: Same as 50 Airframe hours, but with an avionics suite test.
7. 175 Airframe hours: Same as 25 Airframe hours.
8. 200 Airframe hours: Twenty working days for servicing. The rear fuselage is opened up. The engine is also opened and parts tested/replaced. Earlier, the engine would be withdrawn and sent to HAL Koraput. Engine life was subsequently increased to 500 hours from 400 hours. The avionics suite is tested to its limits.
9. 225 Airframe hours: Same as 25 Airframe hours.
10. 250 Airframe hours: Thirty working days for servicing. Ideally, the engine in that aircraft should also be as close to 250 hours as possible. But the poor MTBF of the engine and avionics made this task very difficult. More often than not, the engine would be pulled out and used to replace another due for work. All engines would be sent for overhaul at Koraput at the 250 hour stage.
11. 500 Airframe hours: Aircraft sent for airframe overhaul. HAL takes over the engine.
12. 1000 Airframe hours: Service life of the airframe. This was extended to ~1500 hrs after a major overhaul at HAL. Very few aircraft, however, have crossed 1200 hours.
This is the only aircraft where a fuel tank is carried on the outboard pylon and short/medium range AAMs on the inboard pylon due to stability problems. This required a strong and heavy main wing strut and is one reason the wings were not increased in size, even on the drawing board. The gas flow from the missile affects the airflow into the engine. The relight system comes on at trigger-press, i.e., before the AAM leaves the pylon, to prevent flame out. The engine is no saint. It can cause trouble at any stage and usually does. Such engine snags lead to a delay of, at best, six working days. Since the engine and airframe are married at HAL, they rarely get along together, pun intended. Computerising schedules did help, but not as well as in the case of the Mirage 2000 fleet and, most definitely, the Rafale fleet.